
|
 |
A
fuel cell is a device that generates power by converting
hydrogen into electricity through a chemical reaction. In its
simplest form, fuel cells are just like batteries, except they
do not need to be recharged or replaced - they simply need a
supply of fuel and air. Fuel cells also provide instant power and are a
green energy source with very low to zero emissions.
When looking at a fuel
cell, it is useful to break the system into four distinct parts:
the fuel cell stack, BOP (balance of plant), EMS (energy
management system) and the cartridge. All of these components
operate together to determine the overall size, output and
efficiency of a fuel cell. Different characteristics or
parameters for each of these components can dramatically affect
the overall performance and operation of the fuel cell system.
In fact getting optimal performance from a system is often a
delicate balance of managing air flow and temperature
(BOP), along with algorithms and sensors (EMS) that all affect
the reaction that occurs inside the fuel cell stack.
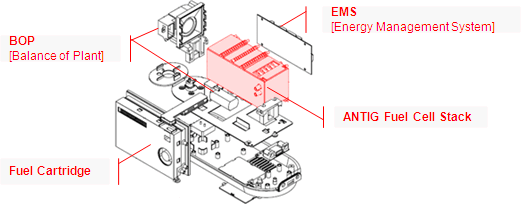
The Fuel Cell Stack
The fuel cell stack is responsible for generating
power. This reaction differs depending on the type of fuel used
by the system. In the case of Antig, Antig stacks are designed
to be used with methanol, which is part of the wood alcohol
family. Inside the stack, there are a number of modules,
each made up of a number of cells. Each cell is part of what is
called a MEA (membrane electrode assembly). MEA's have a
negative side (anode) and a positive side (cathode), and
squeezed between the two is a membrane coated with a special
catalyst. This catalyst
separates each element from the methanol and water, breaking the
hydrogen into positive and negative ions. Once separated, positively charged hydrogen
ions (protons) pass through the fine meshed membrane from the anode side
to the cathode side. The negatively charged hydrogen
ions (electrons) are unable able to pass through this membrane
and are routed around the membrane to the cathode side via an
external circuit. As they travel through this external circuit,
they generate electricity. Once on the cathode side the
electrons are reunited with their partner protons to once again
form hydrogen. At the same time air is passed through the
cathode side which mixes with the hydrogen atoms to form water,
which completes the fuel cell process.
An example of a typical direct methanol fuel cell reaction where
methanol combines with water to produce heat,
in the form of CO2, and hydrogen. After the reaction, hydrogen
mixes with oxygen to form water.
Factors Affecting Fuel Cell
Performance
Fuel cells are designed to
operate in specific types of conditions. For example, oxygen is
a key element required to complete the reaction, as it removes
spent hydrogen from the system as water. If no oxygen is
present, or not in enough quantity, the reaction will
either slow down or stop completely. Also, in direct
direct methanol fuel cells (DMFC), water
management is important as it helps move hydrogen protons
from the anode side across the membrane to the cathode side. If
too much water moves across, then the cathode side can be easily
"flooded'. Not enough water, then the membrane cannot transfer
enough protons to facilitate the reaction. Finally, the fuel used also has an
impact, both on the power of the system as well as it's
operating conditions. In the case of solid oxide fuel cells, the
reaction needs to occur within 600ºC
~ 1000ºC, while methanol systems operate best between 40º ~
80ºC. This balance between air, water
and heat management is both an art and science that ultimately
determines the overall performance of a fuel cell system.
Click here to
learn more about specific types of fuel
cells and their operating conditions.
|